The culmination of several years work by myself and a group of fantastic collaborators was just published in the journal Nature Astronomy (full equivalent preprint version). Several years ago, a colleague at JPL, Dr Jonathan Jiang, presented a seminar about his work on detecting planetary properties using single point reflected light data (original: Jiang et al. 2018, and more recent work: Fan et al. 2019, Gu et al. 2021). By single point reflected light I mean the light reflected by a planet from its star that appears as a single point when viewed from very great distances away. The great distance removes all spatial resolution from what would otherwise be an image if you were looking at the planet from close by. So if you were watching a distant exoplanet for an extended period with a sufficiently powerful and sophisticated instrument, you would see a single point of light that varies in magnitude over time as the planet rotates, moves around its host star, and undergoes its own internal dynamics (such as clouds and their motion). With such an instrument you would likely be able to see these light variations at several wavelengths (sometimes called channels).
Dr Jiang had been using images of Earth from the Deep Space Climate Observatory (DSCOVR) to conduct a special kind of proxy experiment, where the Earth images were disk integrated (removing spatial resolution and converting images to a single pixel) as if the Earth was being viewed from a great distance. The DSCOVR camera being used takes images in 10 different wavelength bands. From the time series of these 10 disk integrated image sets, one can actually deduce many planetary characteristics including orbital period and rotation period. In fact, the great work of Dr Siteng Fan showed that one can even reconstruct spatial features of the planet from non-spatial, multi-wavelength data (see Fig. 4 of Fan et al. 2019).
Following on from this, Dr Jiang posed the following question: could we also use multi-wavelength time series of reflected light to detect life? Around that time I had been discussing various topics in complexity science with friends at the Earth-life Science Institute, and reading some of the incredible work of Prof. Jim Crutchfield and colleagues at UC Davis (this article is a particular favourite: Crutchfield 2012). In this context, a potential answer to Dr Jiang’s question occurred to me: perhaps the presence of life correlates with the complexity of planetary reflectance time series. It is this idea that drove the last ~3 years of research on this project. We had many challenges along the way, and the research would not have been possible without the superb work of Lixiang Gu. Her technique of surface type de and re-composition allowed her to generate many ‘synthetic Earth’ time series with various combinations of surface and cloud types (Gu et al. 2021). It was through analysing these different synthetic Earths that we were able to establish that the statistical complexity metric from computational mechanics, when applied to single point reflectance time series, can actually distinguish qualitative groups of planet types based on their surface features (see Fig. 1 of Bartlett et al. 2022). Further down the line, we were also able to compare Earth time series with Jupiter data from the Cassini mission, thanks to the great work of Jiazheng Li. He was able to extract equivalent time series data for Jupiter in three wavelength channels that are sufficiently close to channels in the original DSCOVR data. After completing the complexity analysis on this new data, we found that Earth’s complexity is approximately 50% higher than Jupiter’s.
So this work highlighted a potential route to quantify planetary complexity using only time series of light reflectance measurements. Advantages of this include the fact that it works for essentially any planetary body, regardless of type or chemistry. It is also completely agnostic to the types of physical, chemical or biological processes that might be occurring on a planet, hence has the potential to detect signatures that might be missed by other techniques that are more focused on life as we know it. At the same time, the method comes with unique challenges, starting with the acquisition of high quality time series for distant exoplanets. I am optimistic that the likes of JWST and the next generation of telescopes will rise to this challenge. Additional challenges include false positives: complex planets that might be completely sterile. We can only make progress on controlling for this possibility by applying our technique to more and more planets (which are in the process of doing). The other side of this is false negatives: simple planets that might contain life. My own opinion is that this is not a huge concern for the following reasons: from what I understand of life, in particular the non-linear characteristics of it, it seems unlikely that it would remain quiescent, hiding in the background of its host planet for extended periods. While I’m sure that early/proto life would be difficult if not impossible to detect from a great distance, after sufficient time (let’s throw out the number of ~billions of years), I would expect most types of life to expand and develop a variety of niches and entangled relationships with its planetary environs (see ideas such as those in: Chopra & Lineweaver 2016 and Lenton et al. 2018).
My hope is that this work introduces many people to ideas from complexity science that might not have otherwise been introduced to them. Epsilon machines and computational mechanics will likely play a key role in the understanding of causal reconstruction from data (as opposed to the pattern recognition one achieves when using a typical machine learning algorithm). Our work is certainly not an absolute answer to the great biosignatures question, but I hope it will play a role among a suite of other methods, which together, eventually, allow us to pinpoint that second data point of lyfe.

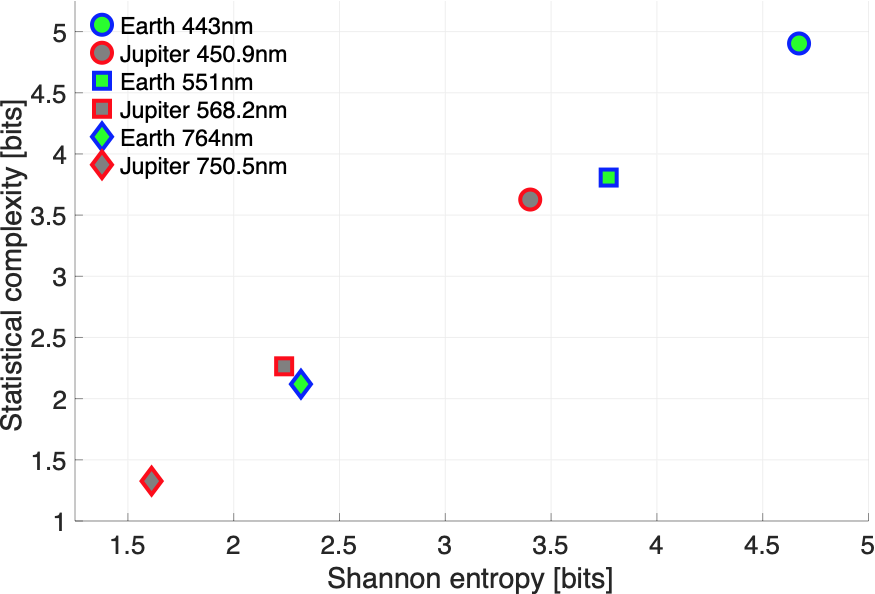